Team:Virginia/Project
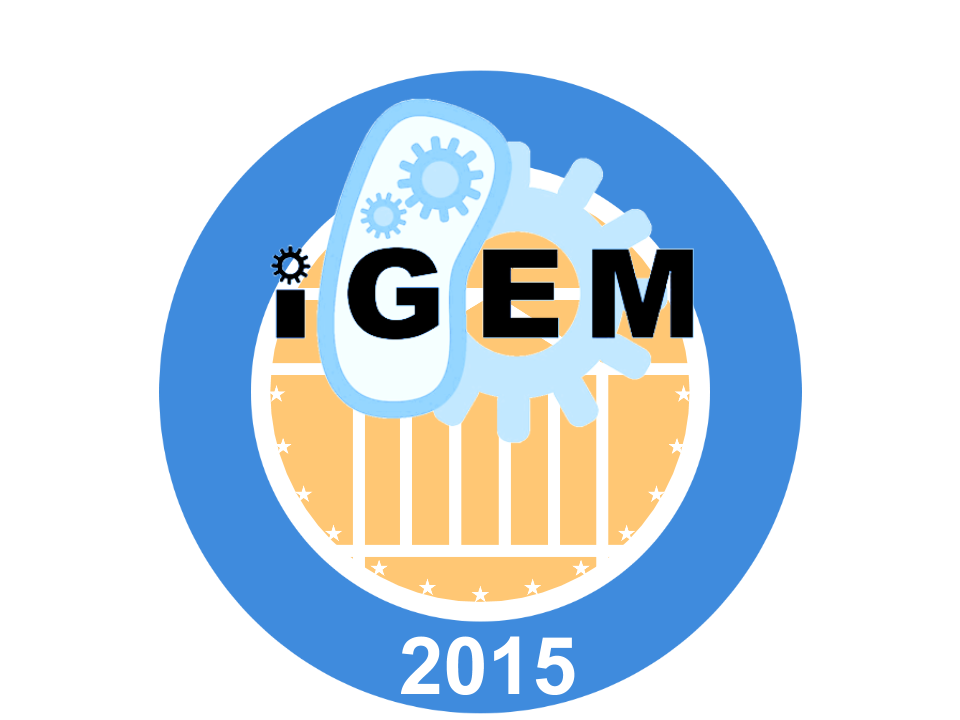
House of Carbs: The Project
(click a tab to reveal more information)
Project Background
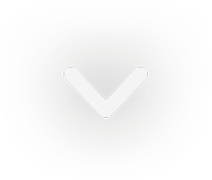
Problem Statement
Diabetes mellitus is a common metabolic disease that is characterized by long-term hyperglycemia, peripheral resistance to human insulin and general lack of insulin production (Kumar et al. 2005). Diabetes is fairly remarkable in its impact mostly due to its far-reaching epidemiological impact; that is, in 2012, nearly 6.9% of American adults had some form of diabetes, which is staggering because this represents a 147% increase in diagnoses over 31 years according to the Centers for Disease Control and Prevention (CDC) (ADA 2014) , (CDC 2014). In addition, there are predictions that by 2050 there will be 552 million cases of diabetes mellitus worldwide (Whiting et al., 2011). The umbrella term “diabetes” covers two distinct disease states, named Type-1 (or juvenile) diabetes mellitus and Type-2 (or acquired) diabetes mellitus. Type-2 diabetes tends to develop as a result of poor dietary habits, alcohol abuse, obesity, or genetic predisposition, and the increase in the incidence of diabetes mellitus as a whole is largely reflective of an increase of the incidence of Type-2 diabetes rather than an increase in juvenile diabetes (ADA 2014).
With regards to Type-2 diabetes (referred to as T2DM) specifically, a number of devastating consequences can arise from increased blood sugar levels on a regular basis, but many of the major complications of T2DM arise from drastic fluctuations in the blood glucose level (Ceriello et al., 2012). Postprandial (post-meal) blood sugar spikes specifically are one of the most damaging complications of diabetes. Many diabetics are able to effectively manage post-meal glycemic spikes with self-administered doses of insulin, but these incidents still kill more Americans per year than any other diabetes-related complications. Arguably, the gravest consequence of glycemic spikes in diabetes patients is the development of progressive macrovascular disease (MVD), which affects the large blood vessels of the body, hardening and blocking these vessels (Ceriello et al. 2012). MVD is the leading cause of death among T2DM patients in the United States, and thus it is a huge target for diabetes treatments research. MVD also frequently leads to other severe complications such as ischemia in the extremities and blindness (Haffner et al., 1998).
Luckily for many T1DM and T2DM patients, it has been shown that the regular control and management of blood glucose levels has been shown to prevent many of the vascular complications of the disease, but most of the time control over glucose is difficult to attain because of the self-dosing insulin treatment system that a lot of moderately to severely sick diabetes patients use is often hard to calibrate and use. Many people with regular hyperglycemia that are not considered diabetic also suffer the risk associated with glycemic spikes and resulting MVD, but these individuals do not have an insulin regimen to regulate high blood sugar levels typically.
The Solution
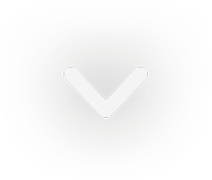
Our solution capitalizes on difference between how simple and complex sugars cause the hyperglycemic spikes.
Simple sugars cause a larger fluctuation in blood glucose, whether it be a sharper spike or even a depression below the homeostatic level, whereas complex sugars do not cause such a large fluctuation, thereby mitigating the spike and lessening the threat to the human body.
Our solution involves engineering a genetically modified E.coli organism into the small intestine of a human where it can hydrolyze simple sugars into complex sugars, thereby mitigating hyperglycemic spikes that occur immediately after meals. Specifically, we studied the mitigation of two simple sugars, fructose and glucose, which constitute a majority of the average American diet in this research.
This solution requires two different BioBricks, a polymerization circuit and an uptake circuit. The uptake circuit, containing the Enzyme-IIA membrane protein, transports glucose into the cytoplasm of the E.coli. The uptake circuit contains the GlgC gene, which codes for BLAH BLAH enzyme, that polymerizes glucose into glycogen. The same circuit contains the SacB gene, which codes for the levansucrase enzyme that hydrolyzes fructose into levan. Levansucrase also confers a fructose sensitivity to the chassis that causes it to lyse after two hours in the presence of 5% sucrose. This releases the hydrolyzed sugars into the small intestine where they are broken down slowly and absorbed into the body, thereby mitigating the hyperglycemic spike.
Synthetic biology is a better solution than existing chemicals, such as alpha-glucosidase inhibitors and analogs of amylin, that perform the same hydrolyses because a bacterium does not require dosage and can easily adapt to variable concentrations of simple sugars present. Therefore, the difficulties of dosage that remain a problem with drugs are not an issue with the bacteria.
Limitations of this solution are mild gastrointestinal symptoms that already exist with chemical solutions due to the similar nature of treatment. Furthermore, the introduction of a probiotic into the body poses a risk of infection or gene transfer into other bacteria of the gut. However, the preferred strain of the E.coli chassis would be a probiotic-certified bacteria that would be biologically harnessed from colonizing the gut or performing horizontal gene transfer.
Sugar Uptake
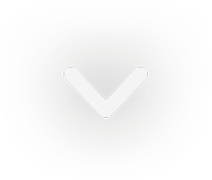
Glucose and fructose are uptaken by different mechanisms into E.coli due to the nature of their polymerization. Glucose, polymerized into glycogen in the cytoplasm, requires to be transferred through the two outer membranes of E.coli. Glucose can diffuse through the outer membrane but requires the use of the Enzyme-IIA to pump it through the inner membrane to the cytoplasm. On the other hand, the levansucrase enzyme polymerizes fructose into levan between the two membranes of E.coli. Fructose, which easily dissolves through the outer membrane but is impermeable to the inner membrane, does not require a protein to transport it to the space between the two membranes where the levansucrase enzyme exists. Therefore, the sugar uptake cassette is only the Enzyme-IIA.
Sugar Polymerization
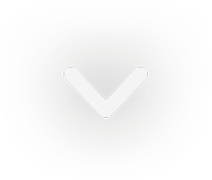
The polymerization plasmid consists of the GlgC gene, which encodes for the protein BLAH that polymerizes glucose into glycogen, and the SacB gene, which encodes for the levansucrase protein that polymerizes fructose to levan and confers levan sensitivity to the chassis in the presence of 5% fructose.
Negative Selection and the Kill-Switch
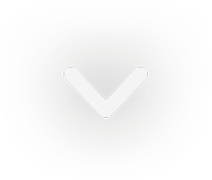
The uniqueness of this kill switch originates from the fact that it is not genetically based and instead relies on the toxic effect of fructose to the chassis. This type of kill-switch better serves our purpose because there is lessened risk of a genetic mutation in the kill-switch gene erasing the kill switch capability. It is less likely that mutations will erase the toxic effects of fructose on E.coli due to the SacB gene.