Difference between revisions of "Team:Waterloo/Modeling/CaMV Replication"
(Revised some bio parts, worked on assumptions some more) |
m (Started with motivation...) |
||
Line 7: | Line 7: | ||
<h2>Motivation</h2> | <h2>Motivation</h2> | ||
<p> | <p> | ||
− | + | Intracellular replication of CaMV may be strongly affected by the addition of CRISPR/Cas9 and in turn will influence viral spread. We were ... | |
</p> | </p> | ||
Revision as of 23:07, 15 September 2015
CaMV Replication
Motivation
Intracellular replication of CaMV may be strongly affected by the addition of CRISPR/Cas9 and in turn will influence viral spread. We were ...
Model Formation
CaMV Genome and Proteins
Overview of Genome
The CaMV genome consists of circular, double-stranded DNA approximately 8000 bp in length . The genome contains three discontinuities typical of pararetroviruses . Discontinuities are repaired within the nucleus to form covalently closed circular (ccc) DNA . There are seven to eight open reading frames (ORFs) which code for six proteins of known function (P1-P6) . Two main RNAs are produced from cccDNA: pregenomic 35S RNA and subgenomic 19S RNA . A third type of transcript, 8S RNA, is also described below, but not incorporated in our model.
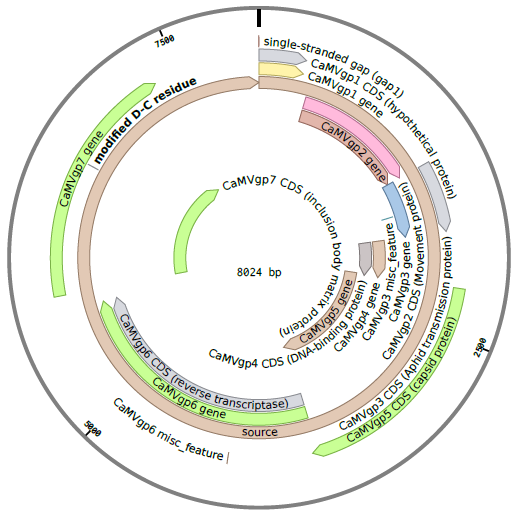
Pregenomic 35S RNA
35S RNA is a complete transcript of the CaMV genome with additional 180 nt terminal repeats . It has two primary functions. The first is to act as an intermediate in genome replication. That is, it's reverse transcribed either in a viral factory or within the capsid to recover gapped dsDNA . It's second function is as polycistronic mRNA coding for P1-P5. In order to produce P1-P5 the 35S promoter must be transactivated by P6 . 35S RNA also undergoes alternative splicing to regulate P1 and P2, which are potentially toxic in high enough concentrations . As such, we account for the relative concentrations of spliced and unspliced 35S RNA in our model since only unspliced 35S RNA will be encapsidated.
Subgenomic 19S RNA
The 19S RNA is a 2.3 kb transcript which contains the ORF for P6 . P6 is constitutively produced from 19S RNA and it's many functions are described in it's own section. Other than producing P6 it has no other function.
8S RNA
8S RNA is 610-680 nt long and is transcribed from the leader sequence of 35S RNA. It's believed that it serves as a decoy for RNA interference, which normally targets the 35S leader . Since it's non-coding and is not targeted by our CRISPR system we do not track 8S RNA in our model.
Movement Protein (P1)
The movement protein (MP) of CaMV facilitates intercellular spread of virions . P1 binds to pectin methyltranferase and ultimately forms intercellular tubules though which virions are transported to neighbouring cells . This protein may also bind cooperatively to RNA to direct 35S and/or 19S RNA to neighbouring cells , which suggests the protein-RNA complex may also move between cells without a capsid. As we are not modelling in detail the transport of virions outside the cell we do not track P1 concentration in our model. Rather, the rate of virions exiting the cell is assumed to be proportional to the number of virions. Additionally, we do not model the possible transport of 35S or 19S RNA transported outside the cell through these tubules.
Aphid Transmission Factor (P2)
The aphid transmission factor (ATF) is a protein which allows for host-to-host transmission of the virus using aphids as vectors. P2 binds to both the aphid stylets and to P3 which allows virions to associate with the stylet upon feeding . It's primary function is host-to-host transmission so we do not track P2 concentration in the cell, but it does produce one electron-lucent inclusion body (elIB) which has minor effects on the system. To form the elIB, P2 associates with microtubules and are transported along the cytoskeleton to a central location. In this process some P3 associates with the P2 and is transported to the elIB . Finally, a small percent (~5%) of virions find their way to the elIB . In this model the amount of P3 and virions which find their way into the elIB are assumed to be negligible.
Virion-Associated Protein (P3)
The virion associated protein (VAP) is a 15 kDa protein is weakly associated with the viral capsid . It's implicated in both host-to-host and cell-to-cell transmission. Due to it's importance in transmission, we track P3 concentration over time and consider a virion "complete" only if it has P3 bound to it.
Capsid Protein (P4)
The capsid protein (CP) is a precursor to numerous other polypeptides which we refer to as P4 subunits. The P4 subunits include P37, P44, P40, P80, P90, and P96, with P80 and P96 being homodimers composed of P37 and P44 respectively . These subunits come together to form a capsid consisting of three layers and using 420 subunits . This model does not capture the complexity of P4 splicing and capsid assembly, rather it assumes packaging is proportional to the amount of P4 available in the cell.
Reverse Transcriptase (P5)
The reverse transcriptase (RT) is a protein which reverse transcribes 35S RNA to form partially double stranded (pds) DNA . The pdsDNA is then encapsidated by P4 subunits to produce virions. It's unknown if reverse transcription occurs within the capsid or in the viroplasm . There is evidence that in Hepatitis B (a similar pararetrovirus) that RT first binds to the pregenome, the RT-RNA complex is recognized by CP to form a capsid, then reverse transcription occurs within the capsid. In this model we assume the reverse transcription and packaging occur simultaneously.
Transactivator/Viroplasmin Protein (P6)
The transactivator/viroplasmin protein (TAV), as it's name suggests, is a protein primarily involved in transactivating the five other proteins and condenses into viroplasms . The viroplasms, aka viral factories or electron-dense inclusion bodies (edIBs), sequester 35S RNA transcripts and produces the other proteins required for viral spread and assembly . The purpose is two-fold: first of all it concentrates the nucleic acid and protein in a smaller volume to increase reaction rates, and secondly it protects the replication machinery from cell defenses . We do not model the formation of edIBs in this model, instead we assume all P6 accumulates as it's produced. This allows us to model the activation of P1-P5.
Overview of Life Cycle
Here we present a brief overview of the CaMV replication cycle, compiled from Khelifa et al. 2010 (), Yasaka et al. 2014 (), Hohn, Fütterera & Hull 1997 (), and Citovsky, Knorr, & Zambryski 1991 ()
- The virion enters the host cell, moves to the nuclear pore, and the viral genome is imported in the nucleus
- Discontinuities in the genomes are repaired to form minichromosomes
- Minichromosomes are used as templates to produce 35S RNA, 19S RNA, and 8S RNA
- P6 is translated from 19S RNA and accumulates in electron-dense inclusion bodies (edIBs)
- P6 transactivates production of P1-P5 from 35S RNA
- Packaging occurs. The exact process is complicated, but either :
- 35S RNA is packaged into virions along with P5 then is reverse transcribed within the capsid, or
- 35S RNA is reverse transcribed in the edIBs before packaging
- The virion may either reinfect the nucleus to increase the copy number of the minichromosome (early in the infection) or it may leave the cell
Due to the complicated nature of viral packaging, we decided in our model to combine the packaging reaction into one term, dependent on P4, P5, and unspliced 35S RNA concentration.
RNA Interference
Someone please update, maybe Laura?
Processes Relevant to our Model
In addition to typical processes such as transcription, constitutive translation, and degradation we must also account for the following processes in our model:
- Virions reinfecting the nucleus
- Repair of pdsDNA
- Splicing of 35S RNA
- RNA interference
- Packaging of 35S RNA
- P6 transactivating production of P1-P5
- Anchoring of P3 to the viral capsid
- Virions exiting the cell
Additionally, since our project uses CRISPR to produce a targeted mutation in the P6 gene, we track both wild-type and mutated versions of genomes, pregenomes, and virions.
Network
DNA
$$\frac{d d_g}{dt} = k_v V (d_{max} - d_{total}) - \alpha_c d_g - k_g d_g - \gamma_d d_g$$ $$\frac{d d_c}{dt} = \alpha_c d_g - k_c d_c - \gamma_d d_c$$ $$\frac{d d_{gm}}{dt} = k_v V_m (d_{max} - d_{total}) - \alpha_c d_{gm} + k_g d_g - \gamma_d d_{gm}$$ $$\frac{d d_{cm}}{dt} = \alpha_c d_{gm} + k_c d_c - \gamma_d d_{cm}$$
RNA
$$\frac{d r_{19S}}{dt} = \alpha_{19S} d_c - (\gamma_{19S}+\gamma_{r}) r_{19S}$$ $$\frac{d r_{35S}}{dt} = \alpha_{35S} d_c - k_p p_4 p_5 f_u r_{35S} - (\gamma_{35S}+\gamma_{r}) r_{35S}$$ $$\frac{d r_{35Sm}}{dt} = \alpha_{35S} d_{cm} - k_p p_4 p_5 f_u r_{35Sm} - (\gamma_{35S}+\gamma_{r}) r_{35Sm}$$
Protein
$$\frac{d p_3}{dt} = \beta_3 \left( \frac{p_6}{p_6+K_6} \right) (r_{35S} + r_{35Sm}) - k_a p_3 (V_i+V_{im}) - \delta_3 p_3$$ $$\frac{d p_4}{dt} = \beta_4 \left( \frac{p_6}{p_6+K_6} \right) (r_{35S} + r_{35Sm}) - k_p p_4 p_5 f_u (r_{35S} + r_{35Sm}) - \delta_4 p_4$$ $$\frac{d p_5}{dt} = \beta_5 \left( \frac{p_6}{p_6+K_6} \right) (r_{35S} + r_{35Sm}) - k_p p_4 p_5 f_u (r_{35S} + r_{35Sm}) - \delta_5 p_5$$ $$\frac{d p_6}{dt} = \beta_6 r_{19S} - \delta_6 p_6$$
Virions
$$\frac{d V_i}{dt} = k_p p_4 p_5 f_u r_{35S} - k_a p_3 V_i$$ $$\frac{d V}{dt} = k_a p_3 V_i - k_v V (d_{max} - d_{total}) - v_e V - \delta_v V$$ $$\frac{d V_{im}}{dt} = k_p p_4 p_5 f_u r_{35Sm} - k_a p_3 V_{im}$$ $$\frac{d V_m}{dt} = k_a p_3 V_{im} - k_v V_m (d_{max} - d_{total}) - v_e V_m - \delta_v V_m$$
Algebraic Equations
$$d_{total} = d_g + d_c + d_{gm} + d_{cm}$$ $$\gamma_r = \frac{L}{1+e^{k (p_6-x_0)}}$$ $$x_0 = \frac{1}{2} p_6 ^{ss}$$ $$p_6 ^{ss} = \frac{\beta_6}{\delta_6} \frac{\alpha_{19}}{\gamma_{19}} d_{max}$$
Assumptions
- Cell concentrations are continuous
- Molecules in the cytosplasm are well-mixed
- No outside infection
- This ODE model only tracks replication within one cell, it cannot track multiple cells. This is handled by the viral spread model instead
- Limited number of genomes in nucleus
- "Another pool of viral genomes, in the order of 10-100 copies of minichromosomes comprising supercoiled circular viral DNA and host histones, accumulates in the nucleus."
- Rate of repair of gapped DNA follows law of mass-action
- Rate of P6 gene mutation is proportional to number of wild-type genomes
- DNA, RNA, proteins, and complete virions degrade, incomplete virions do not
- We assume P3 binds to virions rapidly enough for incomplete virion degradation to be negligible
- We are only targeting 19S RNA
- RNA production follows mass-action
- Concentration of spliced/unspliced RNA is at rapid equilibrium
- Spliced and unspliced 35S RNA degrade at the same rate
- Encapsidation and reverse transcription occur simultaneously
- Information for CaMV is lacking, but for HBV (another pararetrovirus) reverse transcription is initiated during encapsidation and encapsidation is initiated by RT . The complexity of this packaging process is not fully captured in this model
- Only unspliced RNA is packaged
- Spliced RNA lacks P1 and P2 and so, although able to replicate within the cell, virions which package spliced RNA will be unable to propagate
- P1 and P2 do not affect replication dynamics
- P1 is primarily involved in cell-to-cell movement and P2 with host-to-host movement. Neither have a significant impact on the replication process
- P6 is only translated from 19S RNA, translation rate is proportional to cccDNA copy number
- P3, P4, and P5 are translated from 35S RNA, translation is activated by P6
- This is very well established
- All P6 is incorporated in inclusion bodies
- Mass-action anchoring of P3 to virions
- All P4 is instantaneously spliced
- As discussed above the subtleties of encapsidation are not captured by this model
- Virions may reinfect nucleus
- This is very well established
- Virions leave the cell at a constant rate
Parameters
Here are the parameters for the model
Symbol | Value | Units | Description | Source |
---|---|---|---|---|
$k_v$ | 0.1 | min$^{-1}$ | Rate at which virions produced by the cell reinfect the nucleus. | |
$d_{max}$ | 100/vol | molecules/volume | Maximum concentration of viral genomes in the nucleus. | |
$\alpha_c$ | 0.1 | min$^{-1}$ | Rate at which gaps are repaired in gapped DNA to form cccDNA. | |
$k_g$ | 0.01 | min$^{-1}$ | Rate at which the P6 gene on the gapped DNA is modified. | |
$k_c$ | 0.01 | min$^{-1}$ | Rate at which the P6 gene on cccDNA is modified. | |
$\gamma_d$ | 0.001 | min$^{-1}$ | DNA Degradation rate. | |
$\alpha_{19S}$ | 0.01 | min$^{-1}$ | Transcription rate of 19S RNA. | |
$\alpha_{35S}$ | 0.05 | min$^{-1}$ | Transcription rate of 35S RNA. | |
$\gamma_{19S}$ | 0.001 | min$^{-1}$ | Degradation rate of 19S RNA. | |
$\gamma_{35S}$ | 0.001 | min$^{-1}$ | Degradation rate of 35S RNA. | |
$f_u$ | 0.3 | unitless | Fraction of unspliced 35S RNA in the cell (assumed at equilibrium). | |
$\beta_3$ | 0.1 | min$^{-1}$ | Translation rate of P3. | |
$\beta_4$ | 0.1 | min$^{-1}$ | Translation rate of P4. | |
$\beta_5$ | 0.1 | min$^{-1}$ | Translation rate of P5. | |
$\beta_6$ | 0.1 | min$^{-1}$ | Translation rate of P6. | |
$K_6$ | 1000 | molecules/volume | Half-saturation constant for transactivation of P1-P5 production. | |
$\delta_3$ | 0.001 | min$^{-1}$ | Degradation rate of P3. | |
$\delta_4$ | 0.001 | min$^{-1}$ | Degradation rate of P4. | |
$\delta_5$ | 0.001 | min$^{-1}$ | Degradation rate of P5. | |
$\delta_6$ | 0.001 | min$^{-1}$ | Degradation rate of P6. | |
$k_p$ | 0.1 | ??? | Packaging rate. | |
$k_a$ | 0.1 | ??? | Rate of P3 anchoring to virions. | http://www.researchgate.net/publication/7899183_A_coiled-coil_interaction_mediates_cauliflower_mosaic_virus_cell-to-cell_movement |
$v_e$ | 0.1 | min$^{-1}$ | Rate at which virions exit the cell. | |
$\delta_v$ | 0.001 | min$^{-1}$ | Rate of virion degradation. |
Results
Model Validation
Include notes on how the model matches reality/our expectations of reality in this section.