Difference between revisions of "Team:Berlin/senenergene/scenario"
Line 213: | Line 213: | ||
Our product is one that will be used by the industry and is primarily of interest to the state itself, since the government is usually is responsible for the wastewater treatment plants. Our project will therefore develop a public system. A cooperation with the state and the wastewater treatment plants themselves might be achieved, as they seem to be very concerned about the microplastics issue and are trying to find a better solution than what is being used now.<br/> | Our product is one that will be used by the industry and is primarily of interest to the state itself, since the government is usually is responsible for the wastewater treatment plants. Our project will therefore develop a public system. A cooperation with the state and the wastewater treatment plants themselves might be achieved, as they seem to be very concerned about the microplastics issue and are trying to find a better solution than what is being used now.<br/> | ||
Wastewater treatment plants are currently using a very expensive method to filter the microplastics from the sewage water, but it still fails to remove them completely. We believe our filtering unit will offer a better solution for a cheaper price. When in use, the Enzymatic Flagellulose does not need any additional electricity or power to run, so it might even result in lower application costs. Because cellulose itself seems to be highly stable and cannot be degraded easily without enzymes, our product would probably have a good half-life. | Wastewater treatment plants are currently using a very expensive method to filter the microplastics from the sewage water, but it still fails to remove them completely. We believe our filtering unit will offer a better solution for a cheaper price. When in use, the Enzymatic Flagellulose does not need any additional electricity or power to run, so it might even result in lower application costs. Because cellulose itself seems to be highly stable and cannot be degraded easily without enzymes, our product would probably have a good half-life. | ||
− | Finally, having the advantage of being surrounded by Berlin’s vibrant start up and biotech scene, we are currently discussing the potential of our idea with few entrepreneurial academics and business people. This startup could possibly get funded by Bayer. <br/> | + | Finally, having the advantage of being surrounded by Berlin’s vibrant start up and biotech scene, we are currently discussing the potential of our idea with few entrepreneurial academics and business people. This startup could possibly get funded by Bayer. <br/><br/> |
+ | <h5>What are the risks?</h5> | ||
+ | Our modular filter is biocompatible, which means it is environmentally friendly — our product should not affect the environment negatively, nor should it pose a threat to humans since no dangerous compounds are involved. One negative and environmentally harmful aspect might occur during the degradation of microplastics. Since many toxic compounds attach to these particles, the degradation process might set them free at high levels. Also the plastic compounds themselves could turn into environmentally harmful compounds while degradation takes place. | ||
+ | |||
Revision as of 13:56, 18 September 2015
2. Application Scenario
Introduction
Plastic is an environmentally harmful organic polymer that is present everywhere. The production of plastic material often results in the release of CO2, which exacerbates the Greenhouse effect. Within the last five decades, global plastic consumption rose from 5 to 100 million tons per year.[1] Most of this consumption is completely unnecessary and a waste of valuable resources, such as the 600 billion plastic bags that are being redundantly produced annually. Additionally, there is no integrated solid waste management, meaning that plastic waste is neither collected properly nor disposed of in an appropriate manner to avoid the negative impacts on the environment and public health. A good example of this is that out of the produced 14 million tons of Styrofoam only 1% is recycled every year![2] The massive amount of plastic waste that remains unrecycled partly ends up in the oceans. There, it accumulates through two underwater vortexes. One of these plastic accumulations is as big as central Europe and is called “Great Pacific Garbage Patch.” The main problem with this is that plastic cannot be degraded like natural resources. It is very durable and its decomposition can take up to 1000 years.[3] Plastics can be divided into macro- (> 5 mm in diameter) and microplastics (≤ 5 mm in diameter), each of which require different approaches for applications. Macroplastics can be found in plastic bags, bottles, car materials, etc. Microplastics, on the other hand, are present in various everyday products, like peelings and creams, and find their way into the wastewater treatment plants through bathroom drains, and house and industrial sewage. Research is already underway to develop some new techniques to convert macroplastics into fuels without harming the environment. This offers a very promising approach, as the plastic can serve for the production of resources that are in high demand.[4] As for treating microplastics, iGEM Berlin 2015 is constructing a modular filtering machine which can be applied in wastewater treatment plants to degrade microplastics in biodegradable compounds.What’s the problem?
You might be asking, why don’t the wastewater treatment plants filter the plastic? They actually do; however, microplastics are generally separated insufficiently, which is why they enter our natural environment. Consequently, they are taken up by many organisms, including the ones living in rivers, lakes, and the oceans (see Fig. 1), which results in a feeling of satiety that in turn leads to reduced food-intake and, in conclusion, to malnutrition. Also, the uptake of microplastics by organisms causes mechanical injuries, as well as an obstruc¬tion of the digestive system. Toxic compounds, such as DEHP, could leach out from microplastics and affect the flora and fauna of rivers and seas. And even more importantly, microplastics can also affect human beings through the food chain.A research group showed back in 2013 that one microplastic particle smaller than 1 mm can be found per 25 cm3 in depths that range from between about 1000 to 5000 meters. This is a very disturbing fact because the microplastis have reached the very deep sea![5]
How does it work?
Our team is approaching this complex problem by means of synthetic biology using natural products produced by microorganisms to design the Enzymatic Flagellulose, a molecular filtering machine.Our filter consists of a surface made up of cellulose (produced by Acetobacter xylinum) to which bacterial flagella will be immobi¬lized via a cellulose-binding domain. In this case, cellulose itself does not provide any functionality besides acting as a biocompatible carrier. Additionally, it is easy to grow and is a non-plastic material. The single flagellum subunits, also known as flagellin, will be interlinked with plastic degrading enzymes.
Using flagella as a scaffold for enzymes has two major advantages. Firstly, this system enables the creation of a three-dimensional reactive nanostructure that has an increased specific surface with highly catalytic activity. Secondly, flagella may be constructed consisting of various different active sites, which will enable the combination of multiple enzymatic steps in close proximity.

Flagellins from structure to function
Although flagella are an assembly of multiple proteins, the flagellum filament is mostly made of one single protein called flagellin. Flagellin proteins consist of four domains (D0-D3). While the D0 and D1 domains are well conserved, the D2 and D3 domains are highly variable in sequence and length (1: Beatson SA, Minamino T, Pallen MJ. Variation in bacterial flagellins: from sequence to structure. Trends Microbiol. 2006 Apr;14(4):151-5. Epub 2006 Mar 15. PubMed PMID:
Flagella of E. coli – subunits of flagellin https://static.igem.org/mediawiki/2008/0/0f/Ecoli_filament.jpg
This allows us to modify the D3 domain for our purposes by making use of the Synthetic Biology. We designed a flagellin gene (fliC_MCS) with gBLOCKS and introduced specific codons at certain regions of the D3 domain that are supposed to code for the tRNA that will deliver L-Homopropargylglycine (HPG; a non-canonical amino acid). When this gene is introduced into a methionine auxotrophic strain, the bacterium builds in HPG at the certain region of the D3 domain instead of a methionine due to the reason that we do not add methionine to the medium, and the bacterium basically is forced to use HPG. Under mild conditions HPG then reacts to specifically modified arginine residues that we are going to introduce in our enzymes.

Below, you will find the detailed reaction of the degradation of PET by the cutinase which has been worked out by the iGEM Team University of California in 2012:

Degradation of PET by cutinase https://2012.igem.org/Team:UC_Davis/Project/Catalyst
As shown here, the PET is degraded into a component which can be transformed into an intermediate of the citrate cycle and terephthalic acid which’s degradation is still too little known about.
The Cutinase is an α-β enzyme, which has the typical triad structure Ser 120, His 188 and Asp 175. So the active side is composed of three amino acids. The active serine is not buried under surface loops, so it is accessible to solvents. It is composed of a central β-sheet of five parallel strands covered by five helixes on either side of the sheet (Martinez et al., 1992, Fusarium solani cutinase is a lipolytic enzyme with a catalytic serine accessible to solvent). Cutinases are able to hydrolyze the ester-linkage within certain plastics, as Polyethlyeneterephtalate (PET) (C10H804)n.
It could be shown that esterases have a stable pH range from 4,0-8,0, so from acidic to neutral pHs (Faiz et al., 2007, Determination and Characterization of Thermostable Esterolytic Activity from a Novel Thermophilic Bacterium Anoxybacillus genensis A4). Cutinase is an enzyme that once brought to the periplasmic space a leakage occurs that helps to secrete into extracellular matrix. There are several methods to identify the activity of cutinases. One is to incubate small parts of PET within the enzyme, afterwards an analysis by scanning electron microscopy shows the rising porosity of the plastic. Another way is the p-nitrophenol butyrate (pNPB) assay. pNPB, which is a monomer similar to PET, is degraded.
Thereby the absorbance at 405 nm increases. There are different cutinases available for our purpose. Cutinase Hic from Humicola insolens has a temperature optimum at 80°C, which is too high. The cutinase FsC from Fusarium solani ist toxic to E.coli, since our expression is performed in E.coli this cutinase is overruled as well. We decided to use the LC cutinase 2012, since it works at 25°C as good as at 60°C.
Implementation of our product
The Enzymatic Flagellulose unit will be integrated within the secondary wastewater treatment phase, where biological oxidation takes place. While we intend it to be immobilized it on a rotor inside the tanks, another idea might be to use beads and have them flow through the sewage water in the tank. For this decision to be made, we would have to test what geometry has higher efficiencies regarding the degradation of microplastics. That way, the release of microplastics into the environment, its distribution, and related environmental consequences can be prevented. Furthermore, our functionalized matrix can be expanded to other environmental problematic issues, such as removing medicine residues or pesticides from wastewater. Also, after having developed this modular machine, it could be applied directly where wastewater enters the system – meaning in the drain of a home or an industrial facility. For example, we could use it like a sink strainer by making the Enzymatic Flagellulose home compatible. The main reason we began with applying our Enzymatic Flagellulose in the wastewater treatment plants is that the enzymes need some time to carry out the reactions. While there is not enough time if the water just flows through the sink, in the treatment plants it will stay for several hours in one tank (see below). Later, when we have optimized the enzymes to carry out very fast reactions, the implementation of a microplastics degrading sink strainer is the next application of choice.Thus, our modular machine offers a wide range of application possibilities. By varying and combining the interlinked enzymes of different functions, it can be applied diversely and simultaneously for various purposes.
The Wastewater Treatment Plant
Wastewater treatment itself involves four major steps: primary, secondary, tertiary, and post treatment. The primary treatment is a physical process where large debris is separated and sedimented. The separation happens through screening, skimming, and grinding of sewage. In a separate tank, called the settling tank, the sedimentation takes place. There, the organic suspended solids settle to the bottom as the primary sludge and suspended organic matter flows further as the primary effluent.Biological processes, containing oxidation of the primary effluent, are involved in the secondary treatment. These are performed by microorganisms within trickling filters, or aeration tanks called “activated sludge system”. The trickling filter reactor is filled with solid media, like rocks and plastic, on the surface of which bacteria grow. When wastewater trickles down, the bacteria use up the biochemical oxygen demand (BOD) and eventually die, falling off of the media surface. This filter is open to the atmosphere, so that air flows naturally through the media. After the treatment, the water leaves from the bottom of the tank and flows into the secondary settling tank. Bacterial cells settle and are removed from the settling tank as a secondary sludge. Some of the water is recycled to the filter to maintain moist conditions. This is where we envision our Enzymatic Flagellulose will be integrated. The water remains in the secondary settling tank for a couple of hours, which is necessary for the plastic degrading enzymes to have enough time to work.
Another method would be the activated sludge reactor, where the wastewater is aerated in an aeration tank, which allows bacteria to consume BOD and therefore grow, multiply, and flocculate. Here, a high oxygen supply is necessary. The treated water flows into a settling tank, where the bacteria settle. Then, they are removed as the secondary sludge. Part of the sludge is recycled back to the activated sludge tank to maintain bacterial population. The remaining high amount of sludge is anaerobically digested in the post treatment.
In total, the secondary treatment removes carbon, nitrogen, and phosphorus, and the activated secondary sludge is separated from the secondary effluent in a settling tank.
In the tertiary treatment, the effluent is undertaken disinfection by chlorination.
As for the post treatment, the sludge is processed by anaerobic microbial digestion, conditioning, and disposing in an anaerobic digester. Here, the purpose is the stabilization of the organic matter and the reduction of the water content. The latter is for reducing the weight of the sludge and making it more economical to transport to its final disposal. Stabilizing the organic matter prevents the formation of odor and decreases the number of pathogens. The sludge contains cellulose, proteins, lipids, and other insoluble polymers, which are degraded to methane CH4 in four microbial processes: hydrolysis, acidogenesis, acetogenesis, and methanogenesis. In other words, the polymers of the organic matter are first degraded to sugars, amino- and fatty acids and then used for the production of short-chain fatty acids, alcohols, CO2 and H2. These products are used for the formation of acetate, which is followed by the formation of methane CH4.

Overview of the wastewater treatment plant (lecture course TIM at the TU Berlin)
Properties of the Enzymatic Flagellulose
Properties of Cellulose:
A bacterially synthesized cellulose fiber shows exceptional material properties such as high chemical and thermal stability, biocompatibility and bioinertness and high mechanical stability. The diameter of a bacterial cellulose fiber is about 40-60 nm, which corresponds to one-hundredth of the diameter of a plant fiber. The modulus of elasticity is about 134 GPa, which can be compared with the one of cast iron (grey cast iron: E = 90 - 140 GPa). A single fiber shows the tensile strength of 2 GPa, this is comparable with some stainless steel types like AK steel (AK Steel 17-7 PH: Rm = 1,3 - 1,5 GPa).[6],[7],[8],[9]
The study of the iGEM Team of the Imperial College London (2014) advises a maximum working shear stress of 7.5 MPa.[10]
The nano structured system shows a large specific surface (60-100 m2/g), this is responsible for the possibility of intense interactions.[6]
One example is the link of cellulose binding domains (CBDs) to the cellulose matrix by hydropbobic interactions. Genetic engineering is our solution for the attachment of the Flagellas on our surface material. This means an effective immobilization of CBD-flagellin fusion proteins on the cellulose matrix without the need for covalent cross linking. The results of Kauffmann et al. (2000) show that the CBD causes no activity loss of the attached protein. In addition immobilization often results in a higher stability of the protein.[11]
Life-time of the product:
The estimated half-life of cellulose itself at 25° C is about 5-8 million years. It can be degraded aerobically and anaerobically. The degradation is catalyzed by a range of enzymes in cellulolytic microorganisms. Three types of enzymes are involved in the degradation process: endoglucanases, cellobiohydrolases, and β-glucosidases.
The known enzymes responsible for cellulose degradation, as well as the cleavage sites, are shown in table 1.

Table 1: Enzymes responsible for cellulose degradation and cleavage sites. Taken from: http://edoc.hu-berlin.de/dissertationen/schimpf-ulrike-2014-01-31/PDF/schimpf.pdf
Currently, we are still researching some other properties. We are focusing on following questions:
How good are the links between the flagella and cellulose, and the flagella and enzymes?
How does this fact affect the life time of our product?
Scale of production or development:
Synthetic biology is of high interest for maker and do-it-yourself biology community. DIYbio spaces and groups are being formed continuously all over the globe (http://diybio.org/local/). Often people that are involved in diybio are able to come up with clever solutions to manufacture biomaterials as well as bio lab equipment improvising. The production of cellulose in a diybio setting has been demonstrated in various projects like in art projects such as “Xylinum Cones” from Berlin based designer Jannis Huelsen This indicates that, first, there is a communal interest in cellulose as a biomaterial and second that cellulose production does not have to occur in a laboratory setting. For this, the Flagellulose may be further developed outside of the academic setting. Especially so, as the Biobricks produced will be openly accessible through the iGEM registry.
Product on market (business competition):
As the final product does not consist of any GMOs, it won’t be regulated via the German genetic engineering law “Gentechnikgesetz” – a big advantage over other synbio products regarding the marketability of our technique. If in the future it might become obligatory by law for the wastewater treatment plants to reduce the amount of microplastics in their purification processes, our filters would be much in demand.
A competing approach might be the use of microplastics for production of valuable resources.
Location (of production, use and storage): We will produce the Cellulose in Germany, in a S1 laboratory. It can be used worldwide and stored in a cooling room.
Transport: Since the cellulose is relatively robust and the links between our modules stable, there are no worries about transporting our product in different ways.
Funding and business model:
Our product is one that will be used by the industry and is primarily of interest to the state itself, since the government is usually is responsible for the wastewater treatment plants. Our project will therefore develop a public system. A cooperation with the state and the wastewater treatment plants themselves might be achieved, as they seem to be very concerned about the microplastics issue and are trying to find a better solution than what is being used now.Wastewater treatment plants are currently using a very expensive method to filter the microplastics from the sewage water, but it still fails to remove them completely. We believe our filtering unit will offer a better solution for a cheaper price. When in use, the Enzymatic Flagellulose does not need any additional electricity or power to run, so it might even result in lower application costs. Because cellulose itself seems to be highly stable and cannot be degraded easily without enzymes, our product would probably have a good half-life. Finally, having the advantage of being surrounded by Berlin’s vibrant start up and biotech scene, we are currently discussing the potential of our idea with few entrepreneurial academics and business people. This startup could possibly get funded by Bayer.
What are the risks?
Our modular filter is biocompatible, which means it is environmentally friendly — our product should not affect the environment negatively, nor should it pose a threat to humans since no dangerous compounds are involved. One negative and environmentally harmful aspect might occur during the degradation of microplastics. Since many toxic compounds attach to these particles, the degradation process might set them free at high levels. Also the plastic compounds themselves could turn into environmentally harmful compounds while degradation takes place.Thanks to
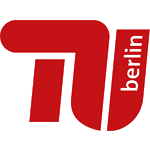














