Difference between revisions of "Team:Berlin/senenergene/scenario"
Line 164: | Line 164: | ||
</strong>Flagella of E. coli – subunits of flagellin https://static.igem.org/mediawiki/2008/0/0f/Ecoli_filament.jpg<strong><br/><br/> | </strong>Flagella of E. coli – subunits of flagellin https://static.igem.org/mediawiki/2008/0/0f/Ecoli_filament.jpg<strong><br/><br/> | ||
This allows us to modify the D3 domain for our purposes by making use of the Synthetic Biology. We designed a flagellin gene (fliC_MCS) with gBLOCKS and introduced specific codons at certain regions of the D3 domain that are supposed to code for the tRNA that will deliver L-Homopropargylglycine (HPG; a non-canonical amino acid). When this gene is introduced into a methionine auxotrophic strain, the bacterium builds in HPG at the certain region of the D3 domain instead of a methionine due to the reason that we do not add methionine to the medium, and the bacterium basically is forced to use HPG. Under mild conditions HPG then reacts to specifically modified arginine residues that we are going to introduce in our enzymes. <br/> <br/> | This allows us to modify the D3 domain for our purposes by making use of the Synthetic Biology. We designed a flagellin gene (fliC_MCS) with gBLOCKS and introduced specific codons at certain regions of the D3 domain that are supposed to code for the tRNA that will deliver L-Homopropargylglycine (HPG; a non-canonical amino acid). When this gene is introduced into a methionine auxotrophic strain, the bacterium builds in HPG at the certain region of the D3 domain instead of a methionine due to the reason that we do not add methionine to the medium, and the bacterium basically is forced to use HPG. Under mild conditions HPG then reacts to specifically modified arginine residues that we are going to introduce in our enzymes. <br/> <br/> | ||
− | <img src="https://static.igem.org/mediawiki/2015/3/30/Team_Berlin_HPG.png"/> | + | <img src="https://static.igem.org/mediawiki/2015/3/30/Team_Berlin_HPG.png"/><br/><br/> |
Below, you will find the detailed reaction of the degradation of PET by the cutinase which has been worked out by the iGEM Team University of California in 2012:<br/> | Below, you will find the detailed reaction of the degradation of PET by the cutinase which has been worked out by the iGEM Team University of California in 2012:<br/> | ||
− | <img src="https://static.igem.org/mediawiki/2015/f/f6/Degradation_of_PET_by_cutinase_UC-Davis.png"/> | + | <img src="https://static.igem.org/mediawiki/2015/f/f6/Degradation_of_PET_by_cutinase_UC-Davis.png"/><br/> |
</strong>Degradation of PET by cutinase https://2012.igem.org/Team:UC_Davis/Project/Catalyst<strong> | </strong>Degradation of PET by cutinase https://2012.igem.org/Team:UC_Davis/Project/Catalyst<strong> | ||
Revision as of 13:16, 18 September 2015
2. Application Scenario
Introduction
Plastic is an environmentally harmful organic polymer that is present everywhere. The production of plastic material often results in the release of CO2, which exacerbates the Greenhouse effect. Within the last five decades, global plastic consumption rose from 5 to 100 million tons per year.[1] Most of this consumption is completely unnecessary and a waste of valuable resources, such as the 600 billion plastic bags that are being redundantly produced annually. Additionally, there is no integrated solid waste management, meaning that plastic waste is neither collected properly nor disposed of in an appropriate manner to avoid the negative impacts on the environment and public health. A good example of this is that out of the produced 14 million tons of Styrofoam only 1% is recycled every year![2] The massive amount of plastic waste that remains unrecycled partly ends up in the oceans. There, it accumulates through two underwater vortexes. One of these plastic accumulations is as big as central Europe and is called “Great Pacific Garbage Patch.” The main problem with this is that plastic cannot be degraded like natural resources. It is very durable and its decomposition can take up to 1000 years.[3] Plastics can be divided into macro- (> 5 mm in diameter) and microplastics (≤ 5 mm in diameter), each of which require different approaches for applications. Macroplastics can be found in plastic bags, bottles, car materials, etc. Microplastics, on the other hand, are present in various everyday products, like peelings and creams, and find their way into the wastewater treatment plants through bathroom drains, and house and industrial sewage. Research is already underway to develop some new techniques to convert macroplastics into fuels without harming the environment. This offers a very promising approach, as the plastic can serve for the production of resources that are in high demand.[4] As for treating microplastics, iGEM Berlin 2015 is constructing a modular filtering machine which can be applied in wastewater treatment plants to degrade microplastics in biodegradable compounds.What’s the problem?
You might be asking, why don’t the wastewater treatment plants filter the plastic? They actually do; however, microplastics are generally separated insufficiently, which is why they enter our natural environment. Consequently, they are taken up by many organisms, including the ones living in rivers, lakes, and the oceans (see Fig. 1), which results in a feeling of satiety that in turn leads to reduced food-intake and, in conclusion, to malnutrition. Also, the uptake of microplastics by organisms causes mechanical injuries, as well as an obstruc¬tion of the digestive system. Toxic compounds, such as DEHP, could leach out from microplastics and affect the flora and fauna of rivers and seas. And even more importantly, microplastics can also affect human beings through the food chain.A research group showed back in 2013 that one microplastic particle smaller than 1 mm can be found per 25 cm3 in depths that range from between about 1000 to 5000 meters. This is a very disturbing fact because the microplastis have reached the very deep sea![5]
How does it work?
Our team is approaching this complex problem by means of synthetic biology using natural products produced by microorganisms to design the Enzymatic Flagellulose, a molecular filtering machine.Our filter consists of a surface made up of cellulose (produced by Acetobacter xylinum) to which bacterial flagella will be immobi¬lized via a cellulose-binding domain. In this case, cellulose itself does not provide any functionality besides acting as a biocompatible carrier. Additionally, it is easy to grow and is a non-plastic material. The single flagellum subunits, also known as flagellin, will be interlinked with plastic degrading enzymes.
Using flagella as a scaffold for enzymes has two major advantages. Firstly, this system enables the creation of a three-dimensional reactive nanostructure that has an increased specific surface with highly catalytic activity. Secondly, flagella may be constructed consisting of various different active sites, which will enable the combination of multiple enzymatic steps in close proximity.

Flagellins from structure to function
Although flagella are an assembly of multiple proteins, the flagellum filament is mostly made of one single protein called flagellin. Flagellin proteins consist of four domains (D0-D3). While the D0 and D1 domains are well conserved, the D2 and D3 domains are highly variable in sequence and length (1: Beatson SA, Minamino T, Pallen MJ. Variation in bacterial flagellins: from sequence to structure. Trends Microbiol. 2006 Apr;14(4):151-5. Epub 2006 Mar 15. PubMed PMID:
Flagella of E. coli – subunits of flagellin https://static.igem.org/mediawiki/2008/0/0f/Ecoli_filament.jpg
This allows us to modify the D3 domain for our purposes by making use of the Synthetic Biology. We designed a flagellin gene (fliC_MCS) with gBLOCKS and introduced specific codons at certain regions of the D3 domain that are supposed to code for the tRNA that will deliver L-Homopropargylglycine (HPG; a non-canonical amino acid). When this gene is introduced into a methionine auxotrophic strain, the bacterium builds in HPG at the certain region of the D3 domain instead of a methionine due to the reason that we do not add methionine to the medium, and the bacterium basically is forced to use HPG. Under mild conditions HPG then reacts to specifically modified arginine residues that we are going to introduce in our enzymes.

Below, you will find the detailed reaction of the degradation of PET by the cutinase which has been worked out by the iGEM Team University of California in 2012:

Degradation of PET by cutinase https://2012.igem.org/Team:UC_Davis/Project/Catalyst
Thanks to
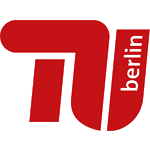














