Difference between revisions of "Team:Nagahama/Experiments"
(→Geraniol production) |
(→Geraniol production) |
||
Line 102: | Line 102: | ||
==Produce of Geraniol and Farnesol== | ==Produce of Geraniol and Farnesol== | ||
===Geraniol production=== | ===Geraniol production=== | ||
− | [[File:アンケートみのる.jpg|200px|thumb|center| | + | [[File:アンケートみのる.jpg|200px|thumb|center|fig8:]] |
− | [[File:アンケート調査グラフ1.jpg|400px|thumb|center| | + | [[File:アンケート調査グラフ1.jpg|400px|thumb|center|fig9:]] |
− | [[File:アンケート調査グラフ2.jpg|400px|thumb|center| | + | [[File:アンケート調査グラフ2.jpg|400px|thumb|center|fig10:]] |
===Farnesol production=== | ===Farnesol production=== |
Revision as of 12:29, 14 September 2015
Contents
Result and Discussion
Confirm antibacterial activity of each volatile substances derived from plant
First, we examined our working hypothesis to “Flavorator” that the volatile gaseous substances from plants’ origin can show either the antibacterial or bacteriostatic activity in a box like “KOZOKO”. The results clearly showed that all the volatile substances of wasabi(Japanese horse radish), rose, garlic and onion had antibacterial properties. In the literatures, wasabi, rose, garlic and onion have antibacterial volatiles, such as allyl isothiocyanete (wasabi), geraniol (rose), allicin (garlic), and lachrymaltory-factor (onion) These antibacterial volatiles are produced after complicated pathways, so for their syntheses, various enzymes are required Then, we searched metabolic pathways in E. coli, in which antibacterial volatiles can be either end products or intermediates. The search hit the geraniol. In E. coli, a precursor of geraniol, geranyl diphosphate (GPP), is synthesized. If we can successfully operate one enzyme to E. coli, it may synthesize geraniol using geranyl diphosphate as a precursor. In this context, we designed our system for establishing the concept of “Flavorator” to build up a brand-new biosynthetic pathways, in which geraniol is produced in the E. coli. In doing so, we transfer the three types of genes listed below to create the hyper-producer E. coli of geraniol.
We examined our working hypothesis to “Flavorator” that the volatile gaseous substances from plants’ origin can show either the antibacterial or bacteriostatic activity in a box like Kozoko.
Fragrance of Garlic
○Protocl here
Garlic grated produced its fragrances to suppress unwanted microbial growth. Left pork(A) didn't change the color. Right pork(B) changed Pink to Brown. We found from this result that fragrance of plants have antibacteril volatiles.
Fragrance of wasabi
Protocl here
Wasabi grated produced its fragrances to suppress unwanted microbial growth. We found from this result that fragrance of plants have antibacteril volatiles.
Fragrance of Rose
Protocl here
Rose grated produced its fragrances to suppress unwanted microbial growth. We found from this result that fragrance of plants have antibacteril volatiles.
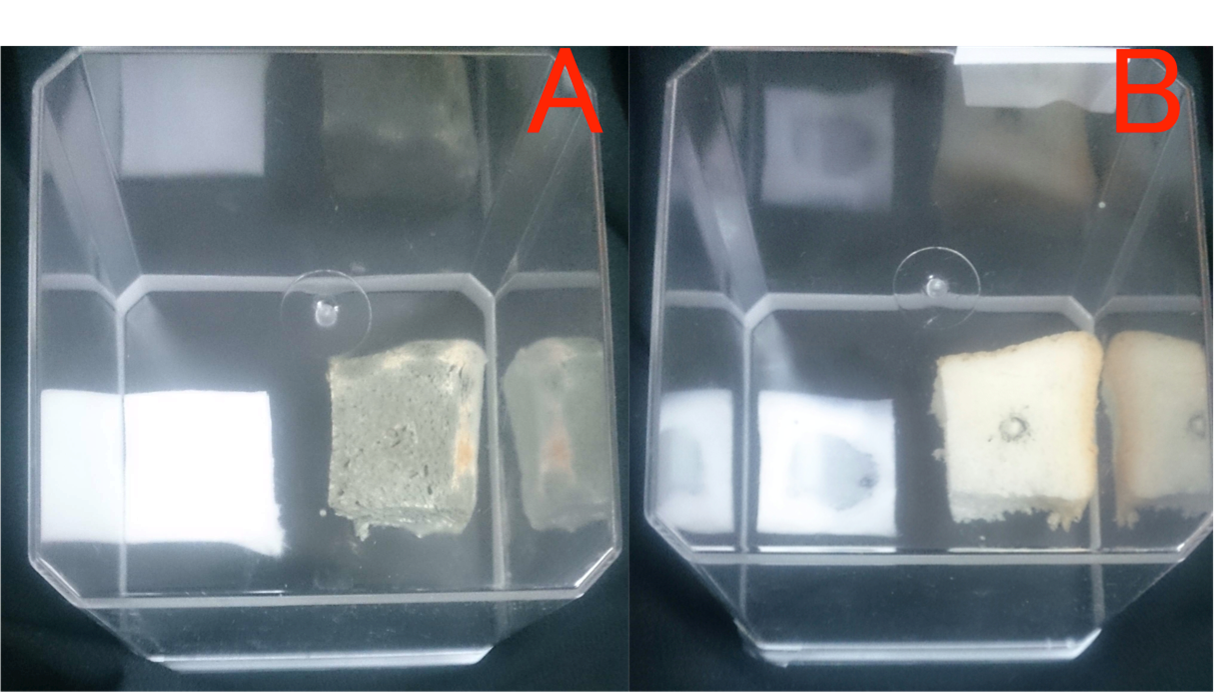
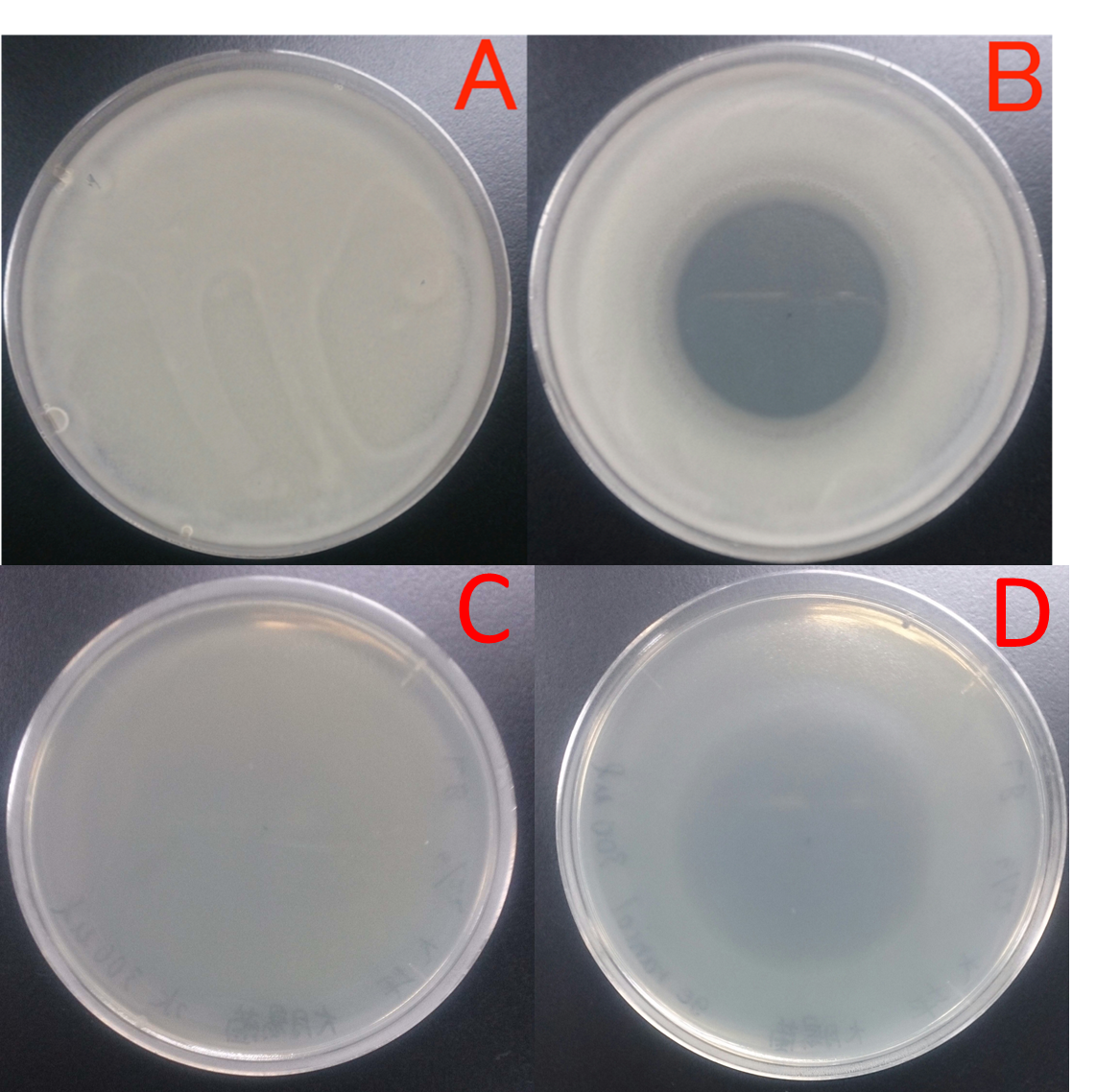
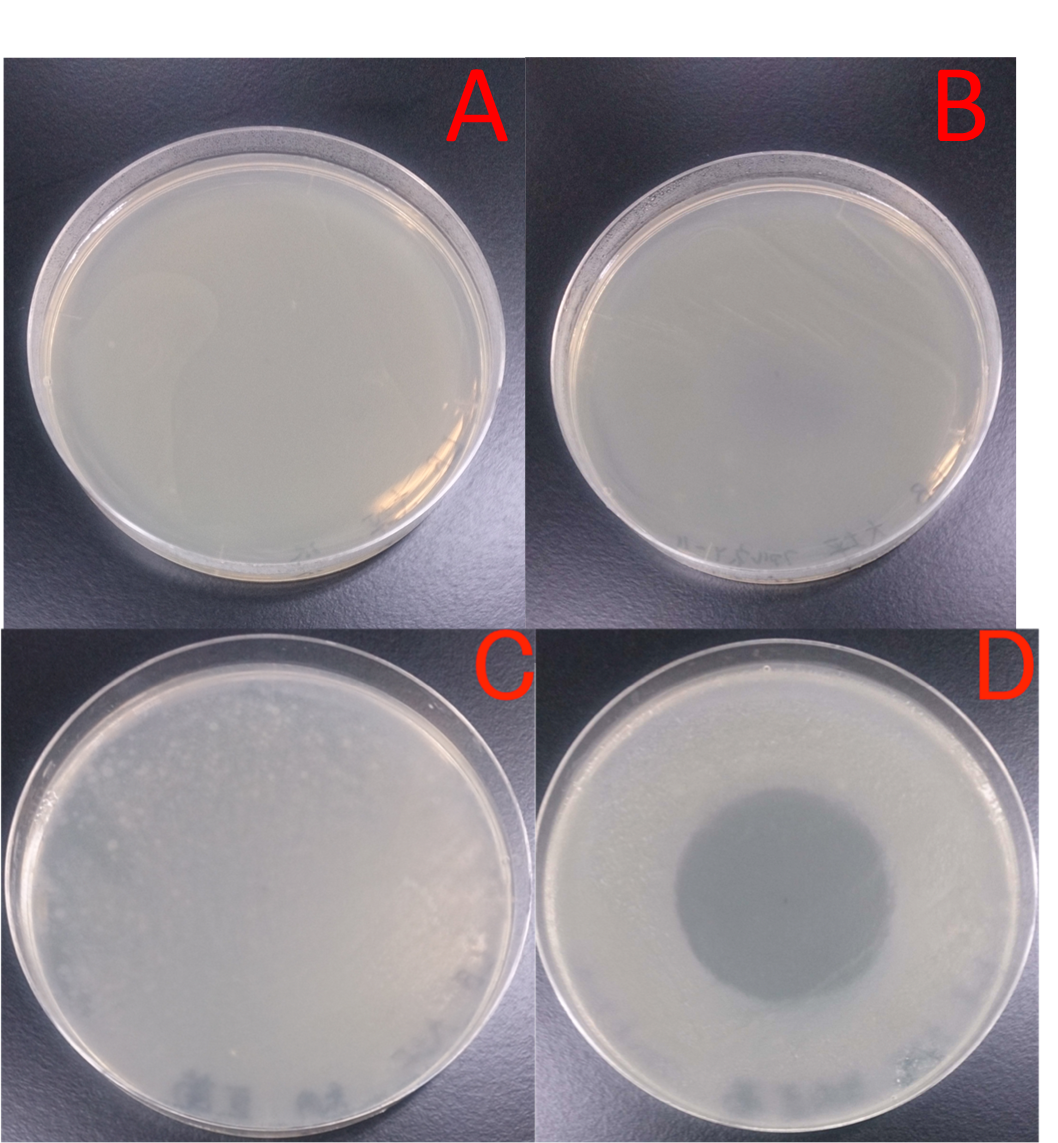
Increase in the amount of terpenoid's precursors
Analysis of ubiquinone-8 synthesized by E. coli JM109/BBa_K1653005
by thin-layer chromatography (TLC)
1. Put E. coli cells (JM109/MEP enzymes) in: methanol (7: 2).
2. Sonicate cells for 30 sec. and cool down 30 sec. and collect supernatants. ( 6 times).
3. Spin down the supernatants at 12,000xg, 10min at 4 ℃, and dry up acetone and methanol.
4. Add 400μl of chloroform : methanol ( 1 : 1 ) to the dried supernatants. Add equal volume 0.7% NaCl and spin down 12,000xg 5min at 4 ℃.
5. Extract the lower layer
6. Add 100μl of chloroform : methanol (2: 1).
7. Spot the extracts on TLC plate and develop extracts using benzene : acetone (7 93).
Produce of Geraniol and Farnesol
Geraniol production
Farnesol production
Farnesol production device [http://parts.igem.org/wiki/index.php?title=Part:BBa_K1653025 (BBa_K1653025)]
λPL+r.b.s.+ispA+MEP+×× (r.b.s.+dxs+r.b.s.+m-idi+r.b.s.+ispDF)
E. coli strain engineered with MEP pathway enzymes, ispDF, idi, and dxs , in combination with the enzyme gens, ispA, produced farnesol (Fig. 4B), which was detected by the Gas chromatography/Mas (Fig. 4A-G), having the same retention time as the farnesol chemica sample (Fig. 4A), while the counterpart control E. coli did not produce farnesol under the same conditions (Fig. 4C). Neither E. coli engineered with MEP pathway enzymes only nor the one engineered ispA only showed any farnesol by the Gas chromatography/Mass (Figs. 4D and E). Farnesol is generated through hydrolysis of farnesyl diphosphate (FPP) by the endogenous phosphatases. Increase in farnesol should be associated with an increased intracellular FPP level. FPP is, in turn, converted from geranyl diphosphate (GPP), whose precursors are IPP and DMAPP. IPP and DMPP are end products of MEP pathway that exists in E. coli. Conversion to FPP from IPP or DMPP requires ispA (or m-ispA). Following this context, we speculate that E. coli could produce farnesol better than the counterpart control cells under the up-regulated cellular conditions of an increased intracellular MEP pathway enzymes by metabolic engineering in combination with the special enzyme that converts IPP or DMAPP into FPP.
Gas Chromatography/Mass(GC/MS)
Fig4:The FOH standard solution (Ref) was used as a control. The peak corresponding to the FOH standard at 8.5 min is indicated by an arrow. The peak at 8.5 min was applied to GC/MS. The FOH standard solution (Ref) was used as a control. E. coli JM109(Bba_K165025) were compared with respect to FOH formation using GC-MS. The fagment patarn is Similar with Ref.
ispA+MEP.dev[http://parts.igem.org/wiki/index.php?title=Part:BBa_K1653025]
λPL+r.b.s.+ispA+MEP+×× (r.b.s.+dxs+r.b.s.+m-idi+r.b.s.+ispDF)
FOH is probably generated through FPP hydrolysis by endogenous phosphatases, which are induced by an increased intracellular FPP level Analogously, we hypothesized that E. coli could produce FOH under cellular conditions of an increased intracellular FPP level through metabolic engineering. A MEP pathway has been shown to synthesize IPP and DMAPP efficiently in E. coli. Because of its high hydrophobicity and low volatility, decane was chosen to extract and solubilize FOH from culture broth. The decane overlay in the two-phase culture did not affect growth, and FOH could be solubilized in the decane phase with negligible volatile loss. We adopt 1 mL of decane overlaid to 5 mL of culture broth. Two-phase culture of E. coli JM109 (BBa_K165025) was carried out in 2YT medium containing 1% glycerol at 29°C for 48 h. The decane phase of the two-phase culture was collected to analyze the FOH content by GC-MS. In the GC-MS analysis (Fig. 4A-G), there was a main peak at 8.5 min in the collected decane phase sample, which corresponded to the reference solution of the standard FOH compound dissolved in decane. Mass spectrometry confirmed that the peak at 8.5 min was FOH (Fig. 4-A). However, the peak was not observed in two-phase culture without introducing BBa_K165025. The formation of FOH from FPP was further confirmed by blocking FPP synthesis. In the GC-MS, the FOH peak was observed in E. coli JM109 (BBa_K165025) culture, whereas no peak was observed with transformed E. coli JM109. It was found that FOH need not only ispA(BBa_K165018) but also MEP(BBa_K165024) in E. coli.We submit new part(BBa_K165025) as producing FOH.
Gas Chromatography/Mass(GC/MS)
Fig4:The FOH standard solution (Ref) was used as a control. The peak corresponding to the FOH standard at 8.5 min is indicated by an arrow. The peak at 8.5 min was applied to GC/MS. The FOH standard solution (Ref) was used as a control. E. coli JM109(Bba_K165025) were compared with respect to FOH formation using GC-MS.
Export of geraniol from E. coli
Export
[http://parts.igem.org/wiki/index.php?title=Part:BBa_K1653020 BBa_K1653020]

In this figure, intracellular content of geraniol was less in the strain E. coli JM109 (marA) than the strain E. coli JM109 (WT). The concentrations of intracellular geraniol from E. coli JM109 (marA) was 42.9 μg/ml, which was 40% lower than that from of E. coli JM109 (WT), 72.2 μg/ml. This figure is suggesting that internalized geraniol could be more efficiently exported through AcrAB-TolC efflux pump following the presumed activation of this gene by introducing the activator marA gene.
Resistance
[http://parts.igem.org/wiki/index.php?title=Part:BBa_K1653020 BBa_K1653020]
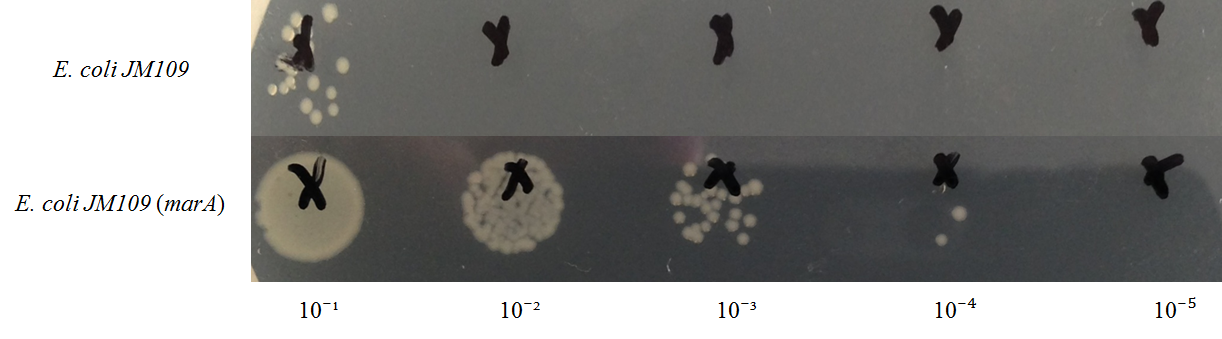
